Atherosclerotic cardiovascular disease is the principal cause of ischaemic heart disease, cerebrovascular disease, and peripheral vascular disease, making it the root of the leading cause of morbidity and mortality worldwide. Atherosclerosis begins with the entrapment and oxidation of low-density lipoprotein (LDL) cholesterol in the arterial endothelium. As a protective response, the endothelium recruits blood monocytes into the arterial wall, which differentiate and mature into active macrophages and engulf toxic oxidized cholesterol products (oxysterols) such as 7-ketocholesterol (7-KC). Although initially protective, this response ultimately leads to atherosclerotic plaque: oxidized cholesterol products accumulate in the macrophage lysosome, and impair the processing and trafficking of native cholesterol and other materials, leading macrophages to become dysfunctional and immobilized in the arterial intima. With ongoing entrapment of oxidized LDL in the tunica intima, more and more of these disabled “foam cells” progressively accumulate in the arterial wall, generating the fatty streaks that form the basis of the atherosclerotic lesion.(1-3)
Rejuvenation biotechnology can be brought to bear against this disease of aging through the identification, modification, and therapeutic delivery of novel lysosomal enzymes derived from microbes to the arterial macrophage — enzymes which are capable of degrading oxidized cholesterol products.(3-8) SENS Foundation-funded researchers have been making steady progress in the identification and characterization of candidate enzymes for several years now,(6-8) and a new report represents a substantial advance in the research: the rescue of cellular oxysterol toxicity by an introduced microbial lysosomal enzyme.
Previous research at the SENS Foundation Research Center and the LysoSENS project centered in Dr. Pedro Alvarez’ lab at Rice University had identified several bacteria that express enzymes capable of degrading 7-KC, mostly in a manner consistent with 3β-oxidation to a ketone. Importantly, the product of such enzymatic transformations exhibited substantially reduced cytotoxicity to human embryonic kidney (HEK) cells relative to the parent molecule;(6) unfortunately, however, there was little reason to expect that these enzymes would function in situ in the human foam cell lysosome, due to its substantially lower pH, high concentration of native proteases, and the derth of required cofactors.
Recently, however, Mathieu et al at Rice took note of a novel cholesterol oxidase from Chromobacterium sp. DS1, which exhibited “significant similarity (53-62%) to the cholesterol oxidases from Burkholderia spp. and Pseudomonas aeruginosa” and a “V(max)/K(m) ratio … higher than those of commercially available cholesterol oxidases”.(11) Relative to other cholesterol oxidases, this enzyme exhibited very high stability in the face of heat, a range of organic solvents, and detergents,(10) suggesting that it might well retain activity in the lysosome. Additionally, the enzyme’s isomeric specificity prevented it from generating stereoisomer product species that permeabilize biological membranes.
These properties commended the Chromobacterium sp. DS1 cholesterol oxidase (DS1 ChOx) to the Rice lysoSENS researchers as a potential candidate for degradation of toxic oxysterols trapped in the foam cell. Few cholesterol oxidases had been tested against 7-KC, and in their hands several bacterial enzymes had failed to oxidize it, or were only capable of doing so at unsuitably-high pH. But in preliminary testing, DS1 ChOx
proved active against both cholesterol and 7KC in detergent or mixed micelles. Overnight incubations of 7KC with DS1 ChOx resulted in complete elimination of 7KC as measured by HPLC (235.8 nm), with concomitant formation of a new product (319 nm). … Enzyme activity against both cholesterol and 7KC increased at higher substrate mole fractions. GC–MS of the HPLC fractions from the DS1 ChOx-catalyzed 7KC reaction revealed the presence of two large peaks, one at 472, which corresponds to the molecular weight of 7KC after derivatization, and the other with a molecular weight of 470 (Fig. 2C). This is supportive of 3β-oxidation, which is expected to result in the loss of two hydrogen atoms.(9)
To be used in vivo to remediate the oxysterol-congested lysosome, however, the enzyme would need to be targeted to, and retain activity in, the endosomal-lysosomal compartment of the cell. Accordingly, Mathieu et al generated a DS1 ChOx construct, utilizing the signal sequence and transmembrane domain of the lysosomal membrane glycoprotein lysosome-associated membrane protein-1 (LAMP-1). After confirming the lysomal localization of their construct when expressed in human fibroblasts, Mathieu et al assessed the ability of the DS1 ChOx/LAMP1 fusion protein to alleviate the cytotoxicity of 7-KC in transfected, 7-KC-treated human fibroblasts. These effects were then compared to several competing approaches, each highlighting a specific property of the enzyme. These included cytoplasmically-targeted DS1 ChOx; overexpression of LAMP-1 alone, without associated DS1 ChOx; sterol 27-hydroxylase, whose metabolites are known to be less toxic that 7-KC itself, but which is expressed in mitochondria rather than at the site of toxicity in the lysosome; and 11β-hydroxysteroid dehydrogenase, which is expressed in the endosome, but whose products are more cytotoxic than the 7-KC substrate itself.(9)
The Right Enzyme in the Right Place
Compared to untreated controls, treatment of fibroblasts with 25, 37.5, 50, or100 μΜ 7-KC resulted in substantial, dose-dependent toxicity, as assayed with the XTT cytotoxicity test. As expected, sterol 27-hydroxylase’s mitochondrial location (being far removed from the lysosomal site of toxicity) meant that it could afford no significant protection against the oxysterol, and 11β-hydroxysteroid dehydrogenase further reduced cell viability, due to the increased toxicity of its reaction products.
By contrast, overexpression of LAMP-1 alone provided significant protection at 37.5 μΜ 7-KC, and possibly in untreated controls, but not at higher concentrations of the oxysterol; the investigators hypothesized that these protective effects may have been due to LAMP-1’s apparent roles in lysosomal stability and cholesterol transport. Similarly, even when confined to the cytosol due to the lack of the signal sequence and LAMP1 transmembrane domain, DS1 ChOx afforded moderate protection against 37.5 or 50 µM 7-KC.
But when targeted to the lysosome, the DS1 ChOx/LAMP1 fusion protein afforded by far the greatest protection against 7-KC, increasing cell viability at 25 µM by 120%, at 37.5 µM by 160%, and also at 50 µM 7-KC, in comparison with mock-transfected cells (Fig. 1)(9).
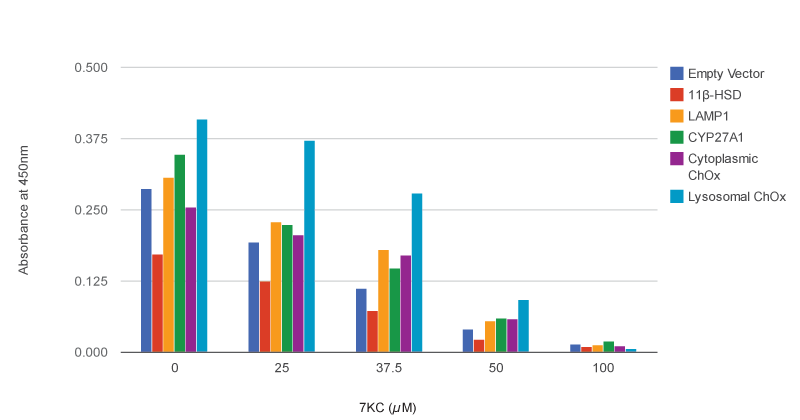
Figure 1. Lysosomally-targeted DS1 Cholesterol Oxidase Protects Against 7-Ketocholesterol Cytotoxicity in Human Fibroblasts. XTT cytotoxicity results following 24 h of transfection with different constructs and exposure to several concentrations of 7-KC. Higher absorbance at 450 nm indicates greater numbers of viable cells. Redrawn from (9) by SENS Foundation volunteer Alex Foster.
Filling in the Blanks
The results, although preliminary, are clearly promising for the potential of lysosomally-targeted DS1 cholesterol oxidase to remediate the oxysterol-intoxicated foam cell, and thus to potentially reverse the root cause of atherosclerosis. They also include some surprises that will need to be probed with further research. Why, for instance, was cell viability improved by DS1 ChOx/LAMP1 fusion protein even in cells that had not been treated with 7-KC (Fig. 1)? One possibility is that in addition to exogenous 7-KC, the enzyme degrades some basal level of oxysterol metabolites that are present even in untreated cells, or that it detoxifies other toxic lysosomal substrates. Another possibility, suggested in personal communication by J Mathieu, is that some metabolite of DS1 ChOx activity might actually provide a benefit to the cell. If such effects also emerge in vivo in any ultimate therapeutic use of suitably-modified DS1 ChOx, they might ultimately provide unexpected additional benefits to the functioning of the aging macrophage lysosome.
Another finding that will need to be investigated further was that DS1 ChOx is actually substantially more active against unmodified cholesterol than it is against 7-KC.(9) This could prove problematic, by perturbing normal cholesterol metabolism or generating bioactive or toxic metabolites. In personal communication, Mathieu notes that the same is true for all of the cholesterol oxidases he has tested, which is logical: bacteria in nature encounter far more unmodified cholesterol than its oxidized products, so natural selection would favor greater specificity for the parent molecule. Indeed, Mathieu writes, “the sterol degrading pathways are fairly conserved. The ideal candidate would be a 7-keto reductase. The bacteria I worked with did reduce the 7-keto group based on metabolites we identified, but the reaction occurs too far downstream. One potential fix is mutagenesis to alter substrate specificity.” Site-specific mutagenesis, or directed evolution using 7-KC or oxidized LDL as the sole substrate for the diversified microbial samples, could be turned to this purpose.
Another important line of investigation will be to test the effects of DS1 ChOx against other oxysterols, and to do so in their true target cell type. 7-KC is generally regarded as the most cytotoxic component of oxidized cholesterol, and the most likely lysosomal toxin, but it is surely not the only one, and the relative toxicity of different oxysterols varies with the experimental system (eg, (11,12)). The Rice LysoSENS group is aware of this, and plans to extend the present results into studies using oxidized LDL, to yield more physiological, hypothesis-neutral, and therapeutically-relevant data on the enzyme’s effects. Similarly, macrophage-like monocytes (such as the THP-1 cell line) and true foam cells behave differently from fibroblasts, HEK cells, or any other cell type; Mathieu will begin working with THP-1 cells shortly, and is working on development of an improved model of the foam cell (personal communication).
With these questions addressed and these properties of the enzyme characterized, later steps would be to replicate such results using enzymes further modified for systemic delivery, testing them first by external application to cultured oxysterol-laden cells in vitro, and later through systemic delivery to animal models of atherosclerosis in vivo, with the ultimate aim of clinical testing in humans with atherosclerotic disease and arterial aging.
Regenerative Medicine Against Atherosclerosis
Medicine has made remarkable progress against cardiovascular disease in recent decades, thanks to antihypertensive drugs and the advent of statins. But cardiovascular diseases remain the greatest cause of death and disease worldwide, and recent years have seen a series of high-profile failures to make further marginal gains over statins alone. Once-promizing agents such as ezetimibe, niacin, ApoA-1 Milano, and torcetrapib have either been found to provide no benefit, or evento increase mortality rates. These results suggest we may be reaching the limits of what can be achieved by pharmacological manipulation of metabolic pathways.
Although preliminary, these early results with the Chromobacterium sp. DS1(9) build on previous progress, carrying forward a clearly-defined developmental plan. If these results can be translated directly into the atherosclerotic artery, at minimum one would expect that the atherosclerotic process would be prevented or arrested. Infiltrating macrophages would for the first time be able to carry out their protective role in the artery, clearing out entrapped oxidized lipids without themselves ultimately falling to lysosomal toxicity and contributing to atherogenesis and the progression of advanced atherosclerotic lesions. Additionally, many ailing macrophages, at risk of becoming necrotic, would be have their functionality returned, their focal adhesions to the intima released, and would migrate out of the core of the lesion.
In an even more optimistic scenario, the abrogation of further insults to the arterial endothelium would be sufficient to allow the body’s instrinsic regenerative capacity to finally catch up with and resolve other aspects of the arterial injury, clearing out the complex secondary pathology of even advanced atherosclerotic lesions and restoring the vessel to health. Aggressive use of statins(14) and other radical lipid-lowering therapies(15) have been demonstrated to shrink atherosclerotic lesions, but lipid-lowering alone has not proven sufficient to address many pathological features of advanced atherosclerotic lesions, including plaque calcification, fibrosis, endothelial erosion, extracellular lipid and lipoprotein deposits, cholesterol crystal formation and retention, and intraplaque neovascularization.(15-20) Moving beyond risk-factor management and into the application of regenerative medicine to repair the damage in the aging artery offers the potential to finally heal the chronic injury of the aging artery.
With this research, SENS Foundation is making progress toward a new kind of therapy, applying the principles of regenerative medicine to the atherosclerotic lesion. This approach actually removes the molecular-level damage that underlies the disease, instead of attempting to perturb metabolism; to reverse the pathological basis of the disease, instead of merely bending the curb of its progression, or reducing the risk of individual cardiovascular events while leaving the disease itself unaddressed. In combination with other rejuvenation biotechnologies (such as the immunotherapeutic clearance of arterial lipoprotein amyloid and the restoration of arterial elasticity), this approach offers the potential to restore aging arteries to youthful health and functionality, allowing them to once again carry the blood of life safely and silently to rejuvenated hearts, muscles, kidneys, and brain.
References
1: Weber C, Noels H. Atherosclerosis: current pathogenesis and therapeutic options. Nat Med. 2011 Nov 7;17(11):1410-22. doi: 10.1038/nm.2538. Review. PubMed PMID: 22064431.
2: Jerome WG, Cox BE, Griffin EE, Ullery JC. Lysosomal cholesterol accumulation inhibits subsequent hydrolysis of lipoprotein cholesteryl ester. Microsc Microanal. 2008 Apr;14(2):138-49. Epub 2008 Mar 3. PubMed PMID: 18312718; PubMed Central PMCID: PMC2837357.
3: Jerome WG. Advanced atherosclerotic foam cell formation has features of an acquired lysosomal storage disorder. Rejuvenation Res. 2006 Summer;9(2):245-55. Review. PubMed PMID: 16706652.
4: de Grey AD. Bioremediation meets biomedicine: therapeutic translation of microbial catabolism to the lysosome. Trends Biotechnol. 2002 Nov;20(11):452-5. PubMed PMID: 12413818.
5: de Grey AD, Alvarez PJ, Brady RO, Cuervo AM, Jerome WG, McCarty PL, Nixon RA, Rittmann BE, Sparrow JR. Medical bioremediation: prospects for the application of microbial catabolic diversity to aging and several major age-related diseases. Ageing Res Rev. 2005 Aug;4(3):315-38. Review. PubMed PMID: 16040282.
6: Schloendorn J, Webb T, Kemmish K, Hamalainen M, Jackemeyer D, Jiang L, Mathieu J, Rebo J, Sankman J, Sherman L, Tontson L, Qureshi A, Alvarez P, Rittmann B. Medical bioremediation: a concept moving toward reality. Rejuvenation Res. 2009 Dec;12(6):411-9. PubMed PMID: 20041735.
7: Mathieu JM, Schloendorn J, Rittmann BE, Alvarez PJ. Medical bioremediation of age-related diseases. Microb Cell Fact. 2009 Apr 9;8:21. PubMed PMID: 19358742; PubMed Central PMCID: PMC2674406.
8: Mathieu J, Schloendorn J, Rittmann BE, Alvarez PJ. Microbial degradation of 7-ketocholesterol. Biodegradation. 2008 Nov;19(6):807-13. Epub 2008 Mar 15. PubMed PMID: 18344006.
9: Mathieu JM, Wang F, Segatori L, Alvarez PJ. Increased resistance to oxysterol cytotoxicity in fibroblasts transfected with a lysosomally targeted Chromobacterium oxidase. Biotechnol Bioeng. 2012 Mar 22. doi: 10.1002/bit.24506. [Epub ahead of print] PubMed PMID: 22447444.
10: Doukyu N, Shibata K, Ogino H, Sagermann M. Purification and characterization of Chromobacterium sp. DS-1 cholesterol oxidase with thermal, organic solvent, and detergent tolerance. Appl Microbiol Biotechnol. 2008 Aug;80(1):59-70. Epub 2008 May 30. PubMed PMID: 18512056.
11: Doukyu N, Shibata K, Ogino H, Sagermann M. Cloning, sequence analysis, and expression of a gene encoding Chromobacterium sp. DS-1 cholesterol oxidase. Appl Microbiol Biotechnol. 2009 Mar;82(3):479-90. Epub 2008 Nov 18. PubMed PMID: 19015844.
12: Rutherford LD, Gieseg SP. 7-ketocholesterol is not cytotoxic to U937 cells when incorporated into acetylated low density lipoprotein. Lipids. 2012 Mar;47(3):239-47. Epub 2011 Nov 29. PubMed PMID: 22124807.
13: Lemaire-Ewing S, Prunet C, Montange T, Vejux A, Berthier A, Bessède G, Corcos L, Gambert P, Néel D, Lizard G. Comparison of the cytotoxic, pro-oxidant and pro-inflammatory characteristics of different oxysterols. Cell Biol Toxicol. 2005 Mar;21(2):97-114. PubMed PMID: 16142584.
14: Nissen SE, Nicholls SJ, Sipahi I, Libby P, Raichlen JS, Ballantyne CM, Davignon J, Erbel R, Fruchart JC, Tardif JC, Schoenhagen P, Crowe T, Cain V, Wolski K, Goormastic M, Tuzcu EM; ASTEROID Investigators. Effect of very high-intensity statin therapy on regression of coronary atherosclerosis: the ASTEROID trial. JAMA. 2006 Apr 5;295(13):1556-65. Epub 2006 Mar 13. PubMed PMID: 16533939.
15: Blankenhorn DH, Hodis HN. George Lyman Duff Memorial Lecture. Arterial imaging and atherosclerosis reversal. Arterioscler Thromb. 1994 Feb;14(2):177-92. Review. PubMed PMID: 8305407.
16: Kovarnik T, Mintz GS, Skalicka H, Kral A, Horak J, Skulec R, Uhrova J, Martasek P, Downe RW, Wahle A, Sonka M, Mrazek V, Aschermann M, Linhart A. Virtual histology evaluation of atherosclerosis regression during atorvastatin and ezetimibe administration: HEAVEN study. Circ J. 2012;76(1):176-83. Epub 2011 Nov 10. PubMed PMID: 22076422.
17: Francis AA, Pierce GN. An integrated approach for the mechanisms responsible for atherosclerotic plaque regression. Exp Clin Cardiol. 2011 Fall;16(3):77-86. PubMed PMID: 22065938; PubMed Central PMCID: PMC3209544.
18: Lewis BS, Halon DA. More progression toward regression? Beyond low-density lipoprotein cholesterol lowering. J Am Coll Cardiol. 2010 Jun 15;55(24):2743-4. PubMed PMID: 20538167.
19: Nasu K, Tsuchikane E, Katoh O, Tanaka N, Kimura M, Ehara M, Kinoshita Y, Matsubara T, Matsuo H, Asakura K, Asakura Y, Terashima M, Takayama T, Honye J, Hirayama A, Saito S, Suzuki T. Effect of fluvastatin on progression of coronary atherosclerotic plaque evaluated by virtual histology intravascular ultrasound. JACC Cardiovasc Interv. 2009 Jul;2(7):689-96. PubMed PMID: 19628194.
20: Moura LM, Ramos SF, Zamorano JL, Barros IM, Azevedo LF, Rocha-Gonçalves F, Rajamannan NM. Rosuvastatin affecting aortic valve endothelium to slow the progression of aortic stenosis. J Am Coll Cardiol. 2007 Feb 6;49(5):554-61. Epub 2007 Jan 22. PubMed PMID: 17276178.