
SENSible Question: Cellular reprogramming turns an old person’s cells young again. So can’t we fix aging by just reprogramming a person’s old cells with reprogramming factors?
This is a tantalizing idea that’s on a lot of our supporters’ minds these days. On the one hand, it’s certainly true that we lose cells with aging and that other cells become dysfunctional. And on the other hand, the cellular reprogramming experiments have in some senses rejuvenated cells in a way that can and should spark excitement — first and foremost, because the technology will greatly enable cell therapy of various kinds, which will be critical to the medical defeat of aging. But the quite rational enthusiasm for a specific technology can sometimes spark a kind of irrational biomedical exuberance so great that even some very prominent geroscientists seem to have begun to fall into a kind of fallacy of composition: the body is made up of cells; therefore, if we rejuvenate all our cells, we will rejuvenate our entire bodies.
People making this intuitive leap are in for an inelegant crash, like a runner in an obstacle course who are so focused on the goal that they both misjudge the height of a hurdle, and don’t see the mudpit that lies directly behind it. By focusing too narrowly on cells and their epigenetic status, they neglect or take too superficial an account of many kinds of cellular and molecular aging damage. We simply are not composed entirely of cells, and replacing lost cells and restoring the original differentiation of cells with epigenetic changes won’t do anything to remove or repair aging damage to the many other functional units that are lost or damaged as we age and that contribute to diseases and disabilities of aging.
Deranged by the Matrix
For one thing, there’s aging damage to the extracellular matrix. The ECM is the lattice of proteins that provide both physical structure and signaling cues for our cells and tissues, and that also have important roles of their own in the body’s movement and plumbing. At the most grossly mechanical level, your quality of life — and your life itself — will be badly impacted if the structured lattice of collagen and other proteins that give form and elasticity to your bones decays or is weakened enough to break under an impact from which a young person would bounce back with no more than a bruise. Similarly, delivering pristine cells to your brain will quickly become a wasted effort if they are subjected to the nonstop pounding of the pulse because your major arteries are still stiff (let alone a if they get hit by a more acute event like a stroke or acute kidney injury, either of which could result from an overly-stiff arterial ECM).
Cells also can’t do their job properly when they are physically impeded from doing so by age changes in the ECM. For instance, aging muscle tissue stiffens, with the result that the muscle is less able to generate eccentric force — even as the resistance also makes more force necessary in order to move one’s joints in the first place. But the stiffening isn’t happening in the muscle fibers themselves: instead, the action of the muscle is impaired in aging by the buildup of abnormally high amounts of ECM around the fibrils. The same thing happens in the aging heart, which impairs the ability of the heart to fully contract and then to relax afterward and refill with blood, contributing to heart failure.
But beyond the ways that damage to aging ECM can physically get in the way of cells doing their jobs, the cells themselves behave abnormally when they are embedded in abnormal ECM. For instance, damage to aging skin proteins impairs their ability to adhere to and provide appropriate mechanical feedback to deep skin cells (fibroblasts), causing fibroblasts to “collapse” and fail to produce new collagen. Age-related loss of a specialized ECM protein called fibronectin causes muscle stem cells to go AWOL, abandoning their “barracks” in the muscle tissue. With fewer muscle stem cells at the ready, injuries to the aging muscle are not fully repaired; conversely, restoring fibronectin levels in aged mouse muscle substantially rejuvenates the aged muscle’s repair capacity. Similarly, the age-related stiffening of the ECM in which muscle stem cells are embedded impairs their ability to reproduce and mature.

Attack of the Amyloids
Conversely, abnormal ECM can enable bad behavior by cancer cells and other abnormal cell types, and cancer cells send out signals that effectively “till the soil” of ECM in distant organs as it begins casting out mobilized cancer cells as “seeds” for the metastasis process that actually kills most cancer patients.
In addition to damage to the ECM, another critical kind of aging damage that would impair the youthful function even of pristine reprogrammed cells is the various extracellular aggregates (“amyloids”) that accumulate outside cells. These are damaged proteins that either physically impede cells’ ability to carry out their function, or cause cellular dysfunction in other ways.
A cardinal example of the former type is cardiac amyloids such as wild-type TTR aggregates, which physically disrupt the architecture of the heart, interfering with its ability to beat. Cardiac amyloidosis is a major driver of some kinds of heart failure in older people, and appears to be almost universal in our longest-lived people at death. A good example of the latter type of amyloid-related cellular dysfunction is when beta-amyloid in its soluble (non-plaque) forms causes the branches through which brain nerve cells communicate with each other to wither away, and also aggravates microglia, turning cells that are meant to protect our neurons into blind agents of destruction. If amyloids are not cleared out, both cells sent in as replacements cells and cells that have undergone partial reprogramming in tissues will still be impaired in their ability to bring us back to health because they will still be subjected to these same assaults.
A Car Needs an Engine to Drive it to the Mechanic
So far, we’ve been thinking about using reprogramming technology either to create replacement cells for those that have been lost to aging processes, or to reprogram cells already in the tissues in order to (as advocates would have it) rejuvenate their function. These applications could in principle deal with cells that are either missing entirely, or that are still present but behaving badly due to reversible changes in their epigenetics — but they can’t do anything about cells that survive, but have suffered certain other kinds of aging damage.
For instance, cells overtaken by mitochondria with large deletion mutations (which are the most problematic kind of mitochondrial damage in aging) almost certainly can’t be restored to normal functioning through reprogramming, for a couple of reasons. First, as we discussed in a previous SENSible Question on mitochondrial mutations, reprogramming technologies require the cell’s DNA to be replicated during the cell cycle, which is why nearly all reprogramming protocols use deep skin cells or immune cells. But with only a couple of unusual exceptions, mitochondria bearing deletion mutations take over in exactly those cell types that can’t dilute them away because they don’t divide, such as brain neurons, heart muscle cells, and muscle fiber segments.
And certainly, reprogramming a cell can’t repair these large deletions, for the simple reason that there is no intact template for the missing mitochondrial genes left in the so-called “homoplasmic” cells that bear them. No one has even reported reprogramming “rho0” cells, which are the closest thing to cells harboring large deletions that can be readily maintained in cell culture.
And contrary to myth, cellular reprogramming doesn’t even repair less severe mitochondrial DNA mutations when they’re present in significant numbers in the cell. Instead, reprogramming leads to cells with different mixtures of the original cell’s intact and mutated mitochondrial genomes, with each reprogrammed cell winding up with different burdens of mutations, in part due to different fractions of healthy and mutated mitochondria persisting after the process is complete. In fact, far from fixing all the mitochondrial mutations present prior to the reprogramming process, a significant number of cells acquire mitochondrial mutations during cellular reprogramming, causing immune rejection when transplanted back into the same person they originally came from!
Damaged Beyond (Epigenetic) Repair
In all probability, the presence of mitochondrial mutations and other aging damage (such as intracellular aggregates, the abnormal splice protein lamin A, and some mutations and epimutations) is one of the main reasons why only a tiny fraction of cells exposed to reprogramming factors ever actually get reprogrammed: about 0.01–0.02% when using the original reprogramming factors, less than 1% with most recent methods, and uniformly less than 5% even in “high-efficiency” methods — and the high-efficiency methods aren’t widely used because they have other problems.
And in addition to not repairing all aging damage, reprogramming itself causes other kinds of damage to some cells that make them useless for rejuvenation biotechnology, such as the newly-created mitochondrial DNA mutations we mentioned above, or abnormal numbers of chromosomes, or the paradoxical mixed bag of reprogramming-induced senescence (RIS).
It’s unsurprising therefore that in many reports, reprogramming efficiency goes down the older the donor gets. Famously, even centenarians’ deep skin cells can be reprogrammed — but getting their cells to do so required additional reprogramming factors that are not needed to reprogram cells from younger donors, and even then, the centenarian’s cells were only reprogrammed as efficiently as those of a 74-year-old. (The finding that there isn’t much difference in the reprogramming efficiency between cells from a 74-year-old and a centenarian doesn’t contradict the general finding of age-related decline, since most people who become centenarians today (and thus without the benefit of rejuvenation biotechnologies) are gifted with a “slow-aging” genes that (by definition) retard the rate of accumulation of aging damage).
This creates a bit of an illusion: because cells bearing the most damage of certain kinds can’t be reprogrammed, many people get an exaggerated sense that the reprogramming process itself has repaired all the aging damage in a cell, when exactly those cells bearing the lowest amount of aging damage to begin with that are most likely to survive and be successfully reprogrammed in the first place.
This is evident when reprogramming cells from (or in) aged donors, including the currently-hot area of “in vivo” partial reprogramming — that is, inducing reprogramming factors directly in the cells in living organisms instead of taking cells from a donor and reprogramming them in a dish. For example, the abstract of a recent report said that such in vivo reprogramming “lead to rejuvenating effects in different tissues, such as the kidney and skin” (my emphasis), which is true as far as it goes — but actually, it only caused meaningful rejuvenating effects in these two tissues, and there are methodological problems about some of the claimed effects.
Around the same time, another study used a drug to activate reprogramming factors in all the cells in middle-aged mice’s bodies — but little actual reprogramming occurred outside of the pancreas, although their activation did lead to other favorable-looking changes in the intestine, stomach, liver, and spleen that did not apparently dependent on actually reprogramming the cells. Contrast that with what the same scientists found in an earlier study, when they activated the same transgenes but in juvenile mice instead of middle-aged adults: at that young age, there was extensive and very complete reprogramming in the pancreas, but also the stomach, intestine, and kidney — so much so that they developed tumors after reprogramming.
And while the scientists who conducted the kidney-and-spleen study had previously reported that reprogramming improved post-injury muscle repair in young animals by enhancing the function of muscle precursor cells, in their more recent report the same group found that it had no such effect in old animals.
Similarly, in another in vivo reprogramming study, which got a lot of advance press via podcast appearances, cellular reprogramming in very young mice allowed for substantial recovery of visual nerve function after their optic nerves were crushed or after artificially-induced glaucoma. However, nearly all of the individual experiments in the report were actually carried out in either extremely young or at worst early middle-aged mice, who by that very fact have relatively low levels of pre-existing aging damage in their cells. And despite the way it was sometimes described in the media (and to an extent even in the published scientific paper), it’s evident that the older (and thus more damaged) the animals were when their visual nerves were injured, the less effective reprogramming was at protecting or restoring their function.
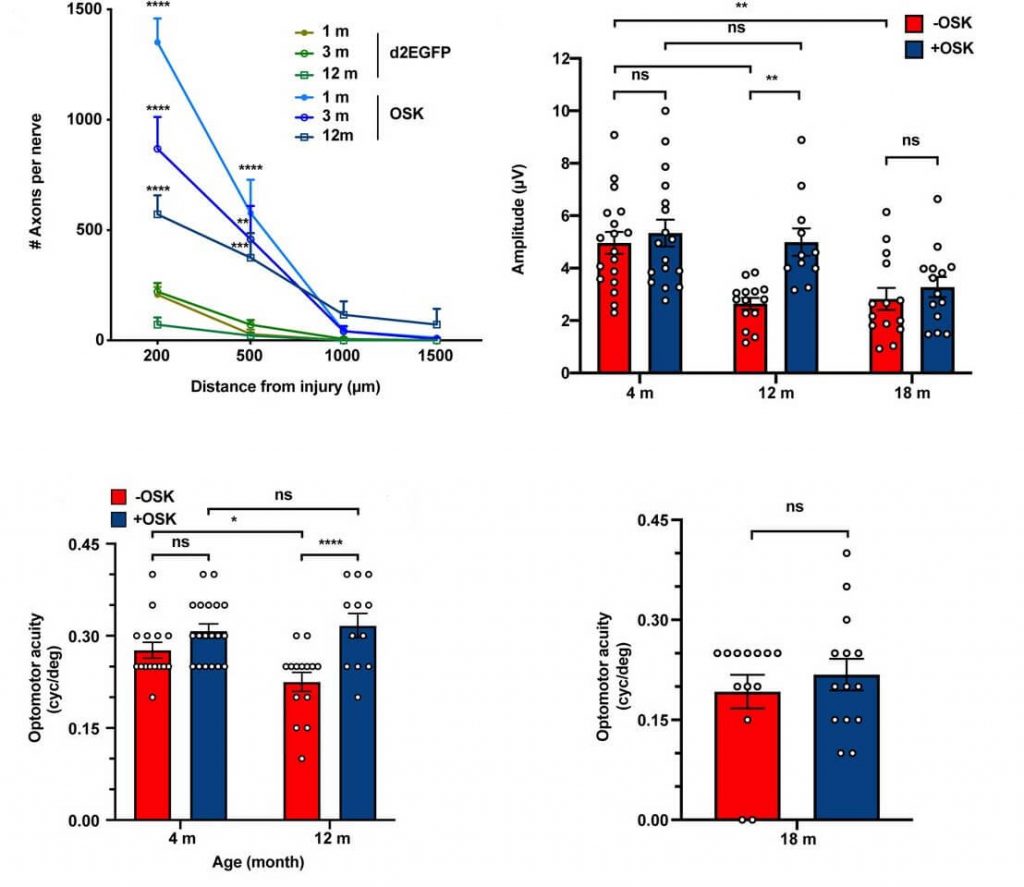
Good Cells Can’t Drive Out Bad
And there are even narrowly cellular forms of aging damage that you can’t or wouldn’t want to “repair” using reprogramming. Yes, you can reverse cellular senescence by reprogramming, and with a few additional tricks you can even reverse reprogramming-induced senescence, but is that a good idea? Remember, the cellular senescence machinery is a kind of emergency brake, which the cell pulls when it is in danger of careening out of control, such as by progressing to become a cancer or by laying down excessive collagen after an injury, leading to fibrosis. Releasing the emergency brake (senescence) on such damaged vehicles (cells) sets it rolling again, making the body once again prone to a crash of one kind or another. (Instead, of course, the solution is to destroy senescent cells).
Indeed, some of these same scientists have previously shown that if they don’t get it quite right, in vivo reprogramming can cause cancers (as seen also here), presumably in some cases because reprogramming takes damaged non-cancerous cells further down the path toward becoming full-blown cancer. (It can also cause cellular changes that quickly kill the in vivo reprogrammed animals for reasons that are unlikely to be related to cancer). This is the main issue that most preoccupies scientists hoping to find a way to harness reprogramming for rejuvenation biotechnology. Fortunately, with some conscious work, there are a number of studies now where no major red flags have appeared — but that may be in part because all such studies have been too short-term for long-term side effects to emerge, and also in part the result of so few cells — and so few types of cells — actually being reprogrammed in these studies.
And reprogramming old but non-cancerous cells into more functional but still non-cancerous cells also isn’t likely to broadly drive out cancer existing, undiagnosed cancer cells, either. That said, an intriguing preliminary study suggest that in at least some cases, it might do something even more useful. In this study, scientists took pancreatic cancer cells from the tumors of pancreatic cancer patients and tried to reprogram them using three different protocols. None of these protocols was able to actually fully reprogram the cells as intended, but the cells that had been partially reprogrammed using their best protocol did display significant changes, including some that are associated with the reprogramming process.
To their own surprise, the researchers found that after this incomplete reprogramming, the cells looked and behave more like normal cells or stem cells in Petrie dish studies, and less like cancer cells. And when they implanted unmodified pancreatic cancer cells into mice, they formed tumors within 8–10 weeks, and all the mice died within three months — but partially-reprogrammed cells didn’t form any tumors within the same three-month study period.
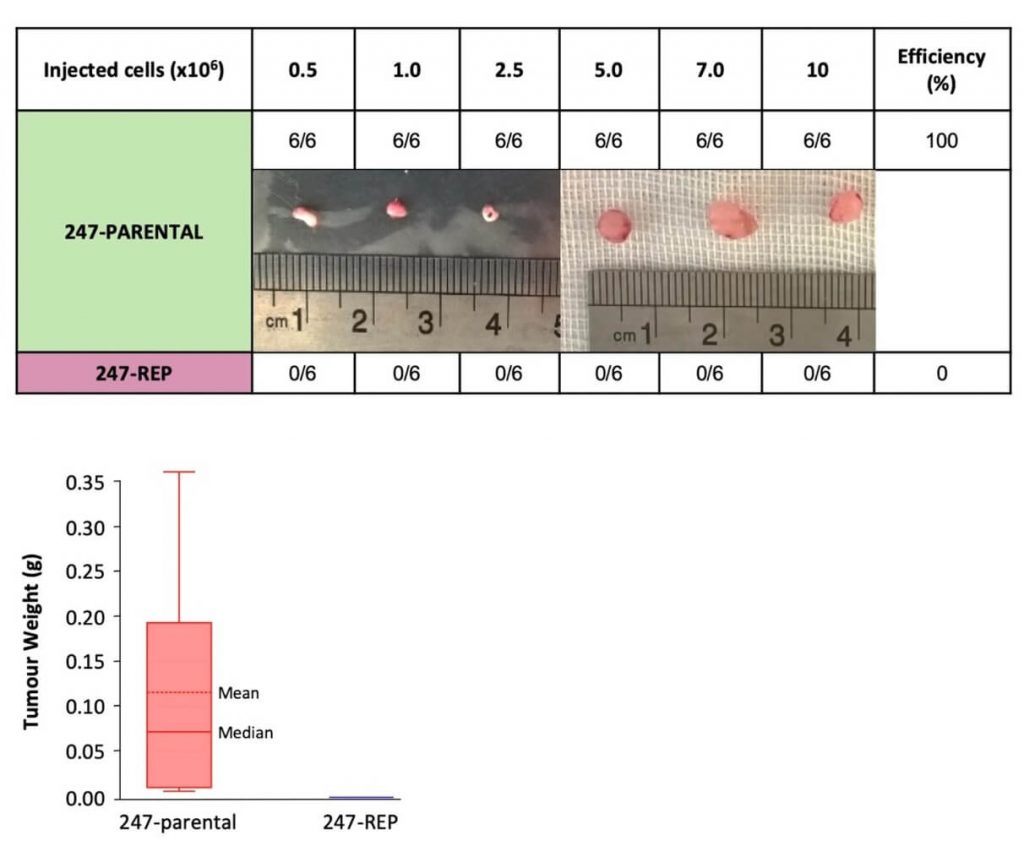
This was a small study, and it was with one particular cancer cell type tested under somewhat artificial conditions. And as we’ve already noted, in vivo reprogramming can cause cancers and abnormal tissue growths if scientists don’t get the dose, duration, and mix of reprogramming factors quite right. So it’s not implausible that the same reprogramming protocol could prevent some nascent cancers from erupting into full-blown disease, while nurturing a dark seed in others.
However this one issue pans out, when we look across the range of cellular and molecular aging damage that we need to remove, repair, or replace in order to free us from the specter of disease and death from aging, we need to spread our chips instead of putting them all into cellular reprogramming as a single inside bet.
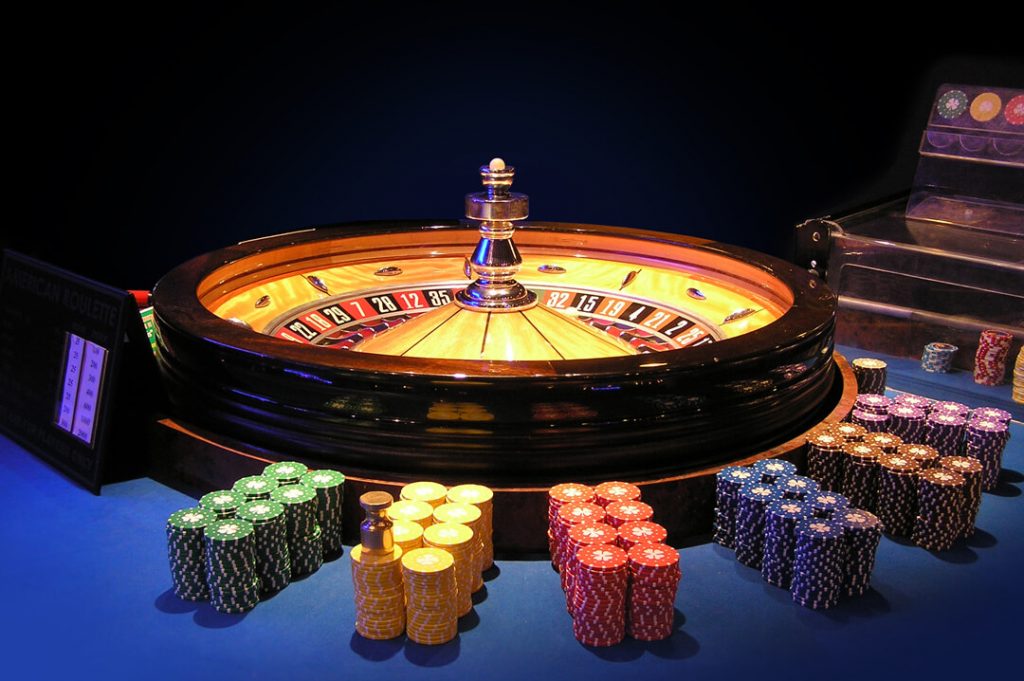