When Dr. de Grey and colleagues proposed ablation of senescent cells (ApoptoSENS) as the “damage-repair” strategy of choice for this kind of aging damage in 2001,[1] you’d’ve been hard-pressed to find the idea even mentioned (let alone advocated) in the scientific literature — and certainly no one was actively working to develop such therapies. And this approach remained largely ignored until 2011, when a powerful proof-of-concept study showed that killing these “zombie cells” using a genetically-engineered “suicide switch” substantially rejuvenated a kind of mouse with a mutation that causes them to accumulate an abnormally high level of these cells.
Soon after that, the Mayo Clinic’s James Kirkland and colleagues developed an ingenious drug-discovery strategy that led to the identification of the first two of a new class of “senolytic” drugs — that is, drugs that selectively destroy senescent cells.[2] Because of their stressful internal environment and the effects of their own secretions, such cells are highly dependent on specific biochemical survival pathways to prevent the activation of their inbuilt “cellular suicide” mechanisms. Senolytic drugs inhibit those pathways, tipping the balance in favor of self-destruction.2,[3]
In the three short years since that initial breakthrough, the progress in ApoptoSENS has been astonishing. A torrent of scientific reports have now shown that ablating senescent cells has sweeping rejuvenative effects — wider-ranging, in fact, than we ourselves had predicted. Drugs and gene therapies that destroy senescent cells can restore exercise capacity,2 lung function,[4] and formation of new blood and immune precursor cells[5] of aging mice to nearly their youthful norms. Senolytic drugs and gene therapies have also ameliorated the side-effects of chemotherapy drugs in mice,[6] and prevented or treated mouse models of diseases of aging such as osteoarthritis;[7] fibrotic lung disease;[8],[9] hair loss;[10] primary cancer[11] and its recurrence after chemotherapy;6 atherosclerosis;[12],[13] and age-related diseases of the heart itself2— as well as preventing Parkinson’s disease[14] and (very recently) frontotemporal dementia,[15],[16] a kind of cognitive aging driven by intracellular aggregates of tau protein, which are also an important driver of Alzheimer’s dementia.
The Need for Smart Weapons
Despite the power of these drugs in animal models, there are still risks associated with them. As noted earlier, senolytic drugs kill senescent cells while sparing normal cells because senescent cells are prevented from collapsing under their self-induced stress by leaning heavily on metabolic pathways that prevent the activation of their in-built cellular suicide (apoptosis) machinery, and/or on other growth and survival pathways. So when these drugs inhibit those same pathways, senescent cells self-destruct, while normal cells not so reliant on these pathways survive.
But while normal cells can handle the inhibition of these pathways when not under stress, they still rely on them when damaged or when the local environment turns hostile: they buy the cells time to hunker down, repair themselves, and in return to normal function when the storm has passed. And some normal cell types are also particularly reliant on some of these pathways. Navitoclax, for instance, which has been used as a senolytic in many studies,5,7,9,13,14 targets a cell-survival factor on which platelets (the cells that allow your blood to clot) are heavily dependent. As a result, Navitoclax was shelved as a cancer therapy despite initial promise, due to cases of dramatic collapses of platelets in some patients and a resulting risk of bleeding to death.[17] And while currently-irreplaceable cells like neurons and heart muscle don’t routinely rely on these pathways, no one wants to lose even a small number of these cells until strong cell therapy is available.
The animal studies of senolytic therapy clearly show that the tradeoff is worth it: that the benefits of purging the aging body of senescent cells far outweigh the dangers of a few lost healthy ones. This is true even in the brain (where killing senescent support cells protects neurons in mouse models of diseases of neurodegenerative aging like Parkinson’s disease14 and tau-driven dementias14,15) and the heart2 (where heart function in aging mice is improved, likely by eliminating senescent cells left over from fibrotic responses to damage in the aging heart). Still, it would be better if there were a way of targeting these drugs more specifically to senescent cells, so that healthy cells wouldn’t be dragged through a trial by fire in order to ensure the elimination of their treacherous neighbors.
And now — at least in mice — there is.
The Calling Card of Senescent Cells
Scientists use a range of different cell markers to identify senescent cells: no one marker is infallible, and different senescence markers are more dominant in different senescent cell types. But the best-established and perhaps most universal sign of all is the activity of an enzyme called senescence-associated beta-galactosidase, or SA-beta-gal. All cells produce SA-beta-gal in their “cellular recycling centers” (lysosomes), but because senescent cells contain an abnormally high number of these organelles, they also produce very high levels of SA-beta-gal — so much so that its activity can be detected under conditions under which it can’t be detected in normal cells.[18]
SA-beta-gal degrades the sugar galactose (one half of the milk sugar lactose), so scientists exploit the overproduction of the enzyme to detect senescent cells using chemically modified forms of lactose that change color when cleaved by the enzyme. But a few years ago, a group of scientists began to wonder if there was a way to take advantage of this property not just to detect senescent cells, but to selectively release drugs that would destroy them.
A Mousetrap With “Cheese” for Senescent Cells
To create a system that would release of cell-destroying drugs selectively in cells with senescent-cell levels of SA-beta-gal, chemists and nanotechnologists working with Drs. José Ramón Murguía and Ramón Martínez-Máñez at the Polytechnic University of Valencia turned to an established platform for the selective delivery of drugs: mesoporous silica nanotubes, or MSNs.[19] These devices are like tiny silicone balls that are neither solid nor simply hollow, but are instead comprised of multiple tiny tubes that come together to form a ball structure, their two ends open at the surface of the ball (Figure 1). The size, shape, and electrical charge of MSNs help determine the tissues in the body in which they accumulate and the cell types that will take them up, and how the cells will handle them once they’re inside. As usually configured, MSNs are taken up by a wide range of cells across the body, which first traffic them to the lysosome and then back out again, releasing them harmlessly back out of the cell.[20],[21]
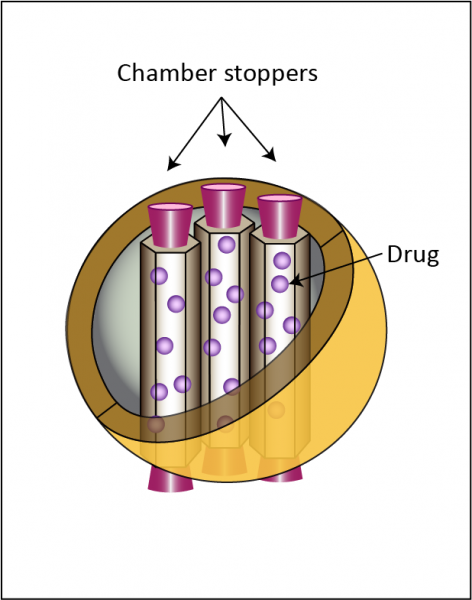
Figure 1. Core features of mesoporous silica nanoparticles (MSN) with “stopper” molecules. Redrawn from ([22]).
But what makes MSNs so useful as drug-delivery systems is that their constituent tubes can be packed with any number of different drugs, and their openings on the surface of the nano-balls “capped” with molecular stoppers that keep the drug sealed inside until the MSN encounters chemical or other conditions that can break open the seal (see Figure 1). So the trick is to identify a molecular stopper that is sensitive to chemical or physical conditions that are found in the type of cell that you want to target, and not found in innocent cells that you want to leave alone. If you can do that, then the MSNs will pass harmlessly through innocent bystander cells with the drug still sealed up inside them — but target cells will recklessly tear the lids off of these nanotechnological Pandora’s boxes, releasing a world of trouble onto themselves.18
As we’ve said, SA-beta-gal’s actual function in the cell is to breaks down the sugar galactose: senescent cells just produce a whole lot more of it than normal cells. So to target MSNs to senescent cells, the Spanish team used galactooligosaccharide (GOS) as the stopper molecule — that is, a series of galactose molecules strung together in a chain. The researchers predicted that with their overabundance of SA-beta-gal, senescent cells would whittle down the chain of galactose molecules until they uncapped the MSNs and released their payload, while the same MSNs would pass through normal cells with their contents still safely sealed up.
In a preliminary study, the grad students working with Murguía and Martínez-Máñez packed a fluorescent dye into MSNs, and then stoppered them with GOS (rho-GOS-MSN), and then watched what happened when they exposed cultures varying in their frequency of senescent cells: a human cell line that had been pushed into senescence by being allowed to replicate under glass until their telomeres ran out; cells taken from patients whose cells turn senescent rapidly because of mutations that prevent them from lengthening their telomeres; and telomerase-active cancer cells, which are called “immortal” precisely because they never run down their telomeres and senesce. As they had hoped, rho-GOS-MSN released their dye into the two senescent cell types, but not in telomerase-active cancer cells, demonstrating the selective release they were looking for.18
Next, to show that GOS-MSN could work in living organisms and not just on cells in a dish, Murguía and Martínez-Máñez’s reached out to biogerontologist Dr. Manuel Serrano at the Spanish National Cancer Institute (CNIO), who has worked extensively on telomere biology and senescence with SENS Research Foundation Research Advisory Board member Dr. Maria Blasco. In a second report, the combined team took tumor-bearing mice and exposed them to DNA-damaging chemotherapy — a treatment that, in mice as in humans, creates a surfeit of senescent cells.6 When they then injected the chemo-treated mice with rho-GOS-MSN, the nanoparticles once again selectively released their dye to label senescent cells, while passing through normal cells without leaving a trace.[23]
Safer, More Effective Cancer Chemotherapy
It’s one thing to label senescent cells with a dye; it’s another thing to kill them — and do so without doing harm to their normal neighbors. To test this, Murguía and Serrano’s team first drove several lines of cancer cells senescent using palbociclib (Ibrance®), a cancer drug that works exactly by shutting down genes that cancer cells require for cell division, thereby driving them into senescence. And this time, they loaded up their GOS-MSN with doxorubicin (AKA Adriamycin®) instead of a dye.[24] Doxorubicin is itself a toxic chemotherapy drug, feared for its potential to damage the hearts of cancer patients that receive it. But for this purpose, its sheer toxicity was useful, because unlike senolytic drugs, doxorubicin is lethal to normal, cancerous, and senescent cells alike. An additional useful feature of doxorubicin is that it’s intrinsically fluorescent, allowing the scientists to easily see where it was realeased.23
GOS-MSN loaded with doxorubicin (DOX-GOS-MSN) passed harmlessly through three non-senescent cancer cell lines, and only released their payload in a small percentage of cells of the same lines that were exposed to palbociclib too briefly to induce widespread senescence (Figure 2(A)). The nanoparticles also had minimal drug release in a fourth line that had been exposed to palbociclib, but which lacks a gene necessary for the drug to induce senescence. But when cancer cells were exposed to palbociclib for long enough to force them into senescence en masse, they lit up with doxorubicin fluorescence (Figure 2(A)), and programmed cell death raged through the population (Figure 2(B)). Meanwhile, pure doxorubicin exhibited no such selectivity, hitting every cell type across the board (Figure 2(A)).
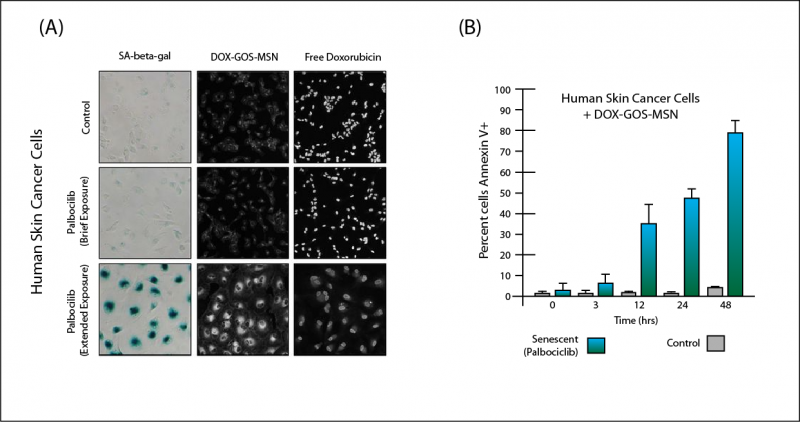
Figure 2. (A): Palbociclib exposure drives cancer cells senescent (left column); DOX-GOS-MSN selectively releases toxic drug into senescent cells (middle column), while doxorubicin alone hits all cell types alike (right-hand column). Adopted from (23), Figure 3(A). (B): DOX-GOS-MSN causes extensive cell death selectively in senescent but not non-senescent cells. Redrawn from (23), Figure 3(B).
The scientists then tried the doxorubicin-loaded GOS-MSN in vivo, in mice that had been implanted with human tumors and then treated with palbociclib. Implanted tumors grew rapidly in untreated mice — and because there were few senescent cells in the tumors, DOX-GOS-MSN alone provided no defense against their growth. Palbociclib alone very substantially slowed the tumor’s growth, implying a massive conversion of formerly rapidly-dividing cancer cells into senescent ones (since that’s how palbociclib fights cancer) (Figure 3).23
By creating that huge mass of cellular zombies, palbociclib gave DOX-GOS-MSN a target at which to shoot. Where palbociclib alone caused tumor growth to slow but not stop, supplementing palbociclib with DOX-GOS-MSN to wipe out the chemo-induced senescent cells led the total tumor mass to shrink away to a smaller size than when either therapy began (Figure 3).23 The researchers got similar results using Navitoclax as the primary cancer therapy: while low-dose Navitoclax has been used successfully as a senolytic in its own right, it was originally intended as a cancer drug before its toxicity to platelets was discovered, and it does work for that purpose at higher doses. And just in case, the researchers repeated experiment with empty GOS-MSN: it had no effect on tumor growth, whether or not the animals had been treated with chemotherapy.23

Figure 3. DOX-GOS-MSN further reduces tumor mass by destroying chemotherapy-associated senescent cells. Redrawn from (23), Figure 3(C).
Murguía and Serrano then looked at another key problem with many otherwise-valuable cancer drugs: toxic side-effects from hitting the wrong cells. As we mentioned earlier, doxorubicin is feared for its potential to ravage heart muscle cells, leading to drug-induced congestive heart failure. And Navitoclax was abandoned as a cancer drug because in addition to killing some kinds of cancer cells, it also caused patients’ clot-forming cells to self-destruct, leading to risk of catastrophic bleeds.
But normal heart muscle cells and platelets are not senescent. So could stoppering these drugs up in GOS-MSN allow them to pass harmlessly through normal heart muscle cells and platelets, but still decimate tumor-associated senescent cells?
Indeed it could! Mice with implanted tumors were first treated with palbociclib, and then with either doxorubicin or Navitoclax — and those, either in “naked” form or sealed up inside GOS-MSN. The two drugs were equally effective at further shrinking the mass of implanted tumor whether they were delivered by direct injection or packaged up in GOS-MSN. But as conventionally delivered, the two drugs caused predictable toxicity to heart muscle cells (DOX) and life-threatening die-off of normal platelets (Nav) (Figure 4). But when packaged up in GOS-MSN, doxorubicin was harmless to the heart (left) and Navitoclax spared most platelets (right) (Figure 4)).23
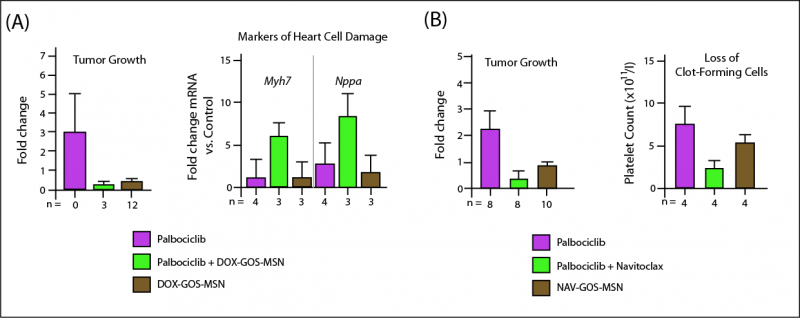
Figure 4. Packaging Doxorubicin (A) and Navitoclax (B) in GOS-MSN shrinks tumor mass by killing tumor-associated senescent cells, but shields healthy cells from deadly drug toxicities. Redrawn from (23), Figure 3(E) and (F).
Breathe Easy
Senescent cells accumulate in the aging lungs, and people suffering with diseases of lung aging suffer an even higher burden of them. Idiopathic pulmonary fibrosis (IPF) is one of these: characterized by scarring of the lung tissue, victims suffer a progressive decline in lung function, making it hard to breathe — both in the sense that it becomes harder to force your lungs to expand to take in air, but also in the sense that your lungs become less and less effective at taking in oxygen and releasing waste CO2. Press a stethoscope up to a patient’s chest, and you can hear a terrible crackling sound inside their lungs when they inhale — like Velcro® being torn apart. There is today only limited therapy: short of lung transplants for a very few, most patients get by on anti-inflammatories, oxygen, and exercises to help them expand their ravaged lungs. Cases often end in death, if nothing else gets them first.
Previous research had already shown that a variety of apoptoSENS strategies can prevent or reverse IPF in mouse models of the disease,8,9 as well as reversing the “normal” loss of lung function with age.4 Murguía and Serrano wanted to see if DOX-GOS-MSN could similarly restore lung function in mice with a model of IPF.23 After first confirming that GOS-MSN distributed evenly across normal and senescent lung tissue but would only release their sequestered dye in tissue with a high senescent cell burden, they treated mice with either straight doxorubicin or DOX-GOS-MSN for two weeks, starting two weeks after inducing model IPF. Lung dysfunction scores remained stubbornly high in animals treated with plain doxorubicin did nothing to help the animals’ lung dysfunction — but DOX-GOS-MSN restored the lung function of the IPF model mice levels equivalent to young mice not subjected to lung damage (Figure 5(A)). DOX-GOS-MSN also reduced the amount of fibrotic tissue in the animals’ lungs, which untargeted doxorubicin was again unable to do (Figure 5(B)).

Figure 5. Doxorubicin targeted to senescent cells by GOS-MSN, but not untargeted doxorubicin, normalize lung dysfunction (A) and greatly reduce lung fibrosis (B) in a mouse model of idiopathic pulmonary fibrosis. Redrawn from (23), Figure 4 (B) and (C).
Senolytic Synergy
As recently as 2015, an announcement like this — the selective targeting of senescent cells in vivo withy a targeted drug strategy, leading to significant functional benefits — would have been breathtakingly exciting news. But after three years of nonstop announcements of novel ApoptoSENS therapeutics and of proofs-of-concept in animal models of one disease of aging after another, it’s human nature to begin taking each new announcement in stride. Yet while its inventors have not stressed it, there is a potential further tweak to the GOS-MSN technology that makes it particularly promising, and that should cause us to pay attention.
As we have gone over a couple of times, prior to GOS-MSN, all senolytic drugs derived their selectivity from the fact that senescent cells lean heavily on active cell-survival pathways to keep themselves alive. Drugs that suppress the activity of these pathways are therefore able to tip the balance over toward programmed cell death in these cells, while sparing most normal cells, which don’t rely on these pathways for their ordinary day-to-day existence.2
But while this is true of normal cells under unstressed conditions, normal cells also rely on those same pathways to carry them through times when they are under stress, and to give them time to recover afterward. Thus, although the net effect of these drugs is undeniably positive, their mechanism of action will necessarily entail occasionally killing off healthy cells in a moment of vulnerability that they could otherwise have survived. This is a particularly important potential risk when the cells in question are not readily replaceable and have to last a lifetime, as is the case with heart muscle cells and brain neurons today.
But what if senolytic drugs were packaged up in GOS-MSN? Existing senolytics expose all cells to their effects, depending on the different metabolic states of senescent and normal cells for their selective killing power. But the selectivity of GOS-MSN particles is different: they work by only releasing their payload of drugs in senescent cells, such that the great majority of normal cells are never exposed to the drugs at all.
And what if rejuvenation biotechnologists took advantage of the strengths of both of these approaches, by loading GOS-MSN with senolytic drugs instead of a generically toxic drug like doxorubicin? You’d expect that this would create a therapy “doubly-selective” for senescent cells: normal cells would almost never be exposed to the drug in the first place — and on the rare occasions when they were, most healthy cells would still escape unscathed, because of the drugs’ intrinsic selectivity for senescent cells.
Conceptually, this combination could lead to greater safety of apoptoSENS therapy, allowing for each round of therapy to use higher doses of drug so as to effect a more thorough clearance of senescent cells, with less risk to normal ones. While the potential has hardly been tested, we’ve already seen a hint of this when Navitoclax was delivered in GOS-MSN in a cancer model: it was just as effective in reducing tumor burden as the “naked” drug, but almost eliminated the loss of platelets that the unpackaged drug entailed (Figure 5(B) above).
Startup Lineup
With those exciting results in hand, Drs. Murguía, Martínez-Máñez, and Serrano have launched a biotech startup to turn GOS-MSN into a human rejuvenation biotechnology. Senolytic Therapeutics (Senolytx) projects that “Designed therapies will be efficacious in treating multiple disorders which are caused and driven by the accumulation of damaged cells. For example, …Idiopathic pulmonary fibrosis (IPF). Also, … cancer cells that have been pushed into senescence by approved chemotherapy and radiotherapy” — that is, exactly the conditions that GOS-MSN treated so successfully in their recent proof-of-concept scientific report.23
And Senolytx is just the latest in a rush of new biotech startups in the senescent cell ablation space, including:
- UNITY Biotechnology: first out of the gate, with the first human patient receiving injections of UBX0101 directly into osteoarthritic knee joints last summer; more senolytics targeted to diseases of the aging eye and lung as well as to systemic sclerosis are expected to follow.
- Oisín Biotechnologies: launched in 2015, with seed funding and access to intellectual property from SENS Research Foundation and our allies at the Methuselah Foundation; they are developing a variation on the “suicide gene” strategy used in the first breakthrough in the field, but which will not permanently alter people’s genomes, can be controlled with a triggering drug, and which avoids other risks associated with virus-based gene therapy.
- Cleara Biotech: working to develop a safer and more effective version of scientific founder Peter de Keizer’s FOXO4-DRI peptide, which triggers cellular suicide in senescent cells by preventing the senescence and apoptosis regulator p53 from binding to FOXO4 (which keeps p53 from initiating the cell’s self-destruct sequence).3
- FoxBio: a joint venture between Foundation alumnus Kelsey Moody’s Ichor Therapeutics and technology financier Jim Mellon’s Juvenescence Limited. FoxBio plans to develop a system for screening for drugs that can prevent FOXO4 or MDM2 from neutralizing the cell-suicide activity of p53. Previous attempts to do such screens have led to unreliable results, because it has been too hard to manufacture full-length, properly folded, bioactive p53 at scale; as a result, companies working to target such interactions have relied on only small fragments of the full protein, or on proteins that do not reflect the complex three-dimensional structure of that p53 adopts our cells. Ichor believes that they can overcome this problem using their proprietary RecombiPure expression technology, and then begin the kind of highly automated screening of large libraries of molecules that could lead to new senoltyics targeting these key interactions.
It must be emphasized that with the exception of FoxBio (who are pursuing a validated target), all of these startups have already proven that their senescent cell ablation therapeutics can selectively clear senescent cells out of the tissues of living, breathing, aging mice — and in so doing, that they rejuvenate tissue function and/or prevent and treat animal models of diseases of aging.
With so many new companies crowding into the space, and with so many potential routes to licensing by the FDA and other regulatory bodies, it now seems not only possible that apoptoSENS therapies will be the first rejuvenation biotechnologies to achieve regulatory approval, but likely that they will be the first to become widely available. Within the decade, people could be taking them as approved drugs for osteoarthritis, IPF, any of several eye diseases — and possibly more common diseases of aging not long afterward, including diabetes, atherosclerosis, lung diseases other than IPF, and as a follow-on therapy for radiation and toxic chemotherapy for cancer.
The real tantalizing outcome here from a SENS perspective is, of course, that when these uses become widely accepted, prophylactic use of senolytics to keep aging people from developing these conditions in the first place could emerge — even as other rejuvenation biotechnologies enter the physician’s armamentarium. In short, the rapid surge of ApoptoSENS type therapies could well be a significant milestone on the road to comprehensive human rejuvenation.
References
- de Grey AD, Ames BN, Andersen JK, Bartke A, Campisi J, Heward CB, McCarter RJM, Stock G. Time to talk SENS: critiquing the immutability of human aging. Ann N Y Acad Sci 2002;959:452-462. PMID: 11976218 [PubMed – indexed for MEDLINE]
- Zhu Y, Tchkonia T, Pirtskhalava T, Gower AC, Ding H, Giorgadze N, Palmer AK, Ikeno Y, Hubbard GB, Lenburg M, O’Hara SP, LaRusso NF, Miller JD, Roos CM, Verzosa GC, LeBrasseur NK, Wren JD, Farr JN, Khosla S, Stout MB, McGowan SJ, Fuhrmann-Stroissnigg H, Gurkar AU, Zhao J, Colangelo D, Dorronsoro A, Ling YY, Barghouthy AS, Navarro DC, Sano T, Robbins PD, Niedernhofer LJ, Kirkland JL. The Achilles’ heel of senescent cells: from transcriptome to senolytic drugs. Aging Cell. 2015 Aug;14(4):644-58. doi: 10.1111/acel.12344. Epub 2015 Apr 22. PubMed PMID: 25754370; PubMed Central PMCID: PMC4531078.
- Baar MP, Brandt RMC, Putavet DA, Klein JDD, Derks KWJ, Bourgeois BRM, Stryeck S, Rijksen Y, van Willigenburg H, Feijtel DA, van der Pluijm I, Essers J, van Cappellen WA, van IJcken WF, Houtsmuller AB, Pothof J, de Bruin RWF, Madl T, Hoeijmakers JHJ, Campisi J, de Keizer PLJ. Targeted Apoptosis of Senescent Cells Restores Tissue Homeostasis in Response to Chemotoxicity and Aging. Cell. 2017 Mar 23;169(1):132-147.e16. doi: 10.1016/j.cell.2017.02.031. PubMed PMID: 28340339; PubMed Central PMCID: PMC5556182.
- Hashimoto M, Asai A, Kawagishi H, Mikawa R, Iwashita Y, Kanayama K, Sugimoto K, Sato T, Maruyama M, Sugimoto M. Elimination of p19(ARF)-expressing cells enhances pulmonary function in mice. JCI Insight. 2016 Aug 4;1(12):e87732. PubMed PMID: 27699227; PubMed Central PMCID: PMC5033852.
- Chang J, Wang Y, Shao L, Laberge RM, Demaria M, Campisi J, Janakiraman K, Sharpless NE, Ding S, Feng W, Luo Y, Wang X, Aykin-Burns N, Krager K, Ponnappan U, Hauer-Jensen M, Meng A, Zhou D. Clearance of senescent cells by ABT263 rejuvenates aged hematopoietic stem cells in mice. Nat Med. 2016 Jan;22(1):78-83. doi: 10.1038/nm.4010. Epub 2015 Dec 14. PubMed PMID: 26657143; PubMed Central PMCID: PMC4762215.
- Demaria M, O’Leary MN, Chang J, Shao L, Liu S, Alimirah F, Koenig K, Le C, Mitin N, Deal AM, Alston S, Academia EC, Kilmarx S, Valdovinos A, Wang B, de Bruin A, Kennedy BK, Melov S, Zhou D, Sharpless NE, Muss H, Campisi J. Cellular Senescence Promotes Adverse Effects of Chemotherapy and Cancer Relapse. Cancer Discov. 2017 Feb;7(2):165-176. doi: 10.1158/2159-8290.CD-16-0241. Epub 2016 Dec 15. PubMed PMID: 27979832; PubMed Central PMCID: PMC5296251.
- Jeon OH, Kim C, Laberge RM, Demaria M, Rathod S, Vasserot AP, Chung JW, Kim DH, Poon Y, David N, Baker DJ, van Deursen JM, Campisi J, Elisseeff JH. Local clearance of senescent cells attenuates the development of post-traumatic osteoarthritis and creates a pro-regenerative environment. Nat Med. 2017 Jun;23(6):775-781. doi: 10.1038/nm.4324. Epub 2017 Apr 24. PubMed PMID: 28436958; PubMed Central PMCID: PMC5785239.
- Schafer MJ, White TA, Iijima K, Haak AJ, Ligresti G, Atkinson EJ, Oberg AL, Birch J, Salmonowicz H, Zhu Y, Mazula DL, Brooks RW, Fuhrmann-Stroissnigg H, Pirtskhalava T, Prakash YS, Tchkonia T, Robbins PD, Aubry MC, Passos JF, Kirkland JL, Tschumperlin DJ, Kita H, LeBrasseur NK. Cellular senescence mediates fibrotic pulmonary disease. Nat Commun. 2017 Feb 23;8:14532. doi: 10.1038/ncomms14532. PubMed PMID: 28230051; PubMed Central PMCID: PMC5331226.
- Pan J, Li D, Xu Y, Zhang J, Wang Y, Chen M, Lin S, Huang L, Chung EJ, Citrin DE, Wang Y, Hauer-Jensen M, Zhou D, Meng A. Inhibition of Bcl-2/xl With ABT-263 Selectively Kills Senescent Type II Pneumocytes and Reverses Persistent Pulmonary Fibrosis Induced by Ionizing Radiation in Mice. Int J Radiat Oncol Biol Phys. 2017 Oct 1;99(2):353-361. doi: 10.1016/j.ijrobp.2017.02.216. Epub 2017 Mar 4. PubMed PMID: 28479002.
- Yosef R, Pilpel N, Tokarsky-Amiel R, Biran A, Ovadya Y, Cohen S, Vadai E, Dassa L, Shahar E, Condiotti R, Ben-Porath I, Krizhanovsky V. Directed elimination of senescent cells by inhibition of BCL-W and BCL-XL. Nat Commun. 2016 Apr 6;7:11190. doi: 10.1038/ncomms11190. PubMed PMID: 27048913; PubMed Central PMCID: PMC4823827.
- Baker DJ, Childs BG, Durik M, Wijers ME, Sieben CJ, Zhong J, Saltness RA, Jeganathan KB, Verzosa GC, Pezeshki A, Khazaie K, Miller JD, van Deursen JM. Naturally occurring p16(Ink4a)-positive cells shorten healthy lifespan. Nature. 2016 Feb 11;530(7589):184-9. doi: 10.1038/nature16932. Epub 2016 Feb 3. PubMed PMID: 26840489; PubMed Central PMCID: PMC4845101.
- Roos CM, Zhang B, Palmer AK, Ogrodnik MB, Pirtskhalava T, Thalji NM, Hagler M, Jurk D, Smith LA, Casaclang-Verzosa G, Zhu Y, Schafer MJ, Tchkonia T, Kirkland JL, Miller JD. Chronic senolytic treatment alleviates established vasomotor dysfunction in aged or atherosclerotic mice. Aging Cell. 2016 Oct;15(5):973-7. doi: 10.1111/acel.12458. Epub 2016 Aug 5. PubMed PMID: 26864908; PubMed Central PMCID: PMC5013022.
- Childs BG, Baker DJ, Wijshake T, Conover CA, Campisi J, van Deursen JM. Senescent intimal foam cells are deleterious at all stages of atherosclerosis. Science. 2016 Oct 28;354(6311):472-477. Epub 2016 Oct 27. PubMed PMID: 27789842; PubMed Central PMCID: PMC5112585.
- Chinta SJ, Woods G, Demaria M, Rane A, Zou Y, McQuade A, Rajagopalan S, Limbad C, Madden DT, Campisi J, Andersen JK. Cellular Senescence Is Induced by the Environmental Neurotoxin Paraquat and Contributes to Neuropathology Linked to Parkinson’s Disease. Cell Rep. 2018 Jan 23;22(4):930-940. doi: 10.1016/j.celrep.2017.12.092. Epub 2018 Jan 28. PubMed PMID: 29386135; PubMed Central PMCID: PMC5806534.
- Bussian TJ, Aziz A, Meyer CF, Swenson BL, van Deursen JM, Baker DJ. Clearance of senescent glial cells prevents tau-dependent pathology and cognitive decline. Nature. 2018 Oct;562(7728):578-582. doi: 10.1038/s41586-018-0543-y. Epub 2018 Sep 19. PubMed PMID: 30232451; PubMed Central PMCID: PMC6206507.
- Musi N, Valentine JM, Sickora KR, Baeuerle E, Thompson CS, Shen Q, Orr ME. Tau protein aggregation is associated with cellular senescence in the brain. Aging Cell. 2018 Aug 20:e12840. doi: 10.1111/acel.12840. [Epub ahead of print] PubMed PMID: 30126037.
- Debrincat MA, Pleines I, Lebois M, Lane RM, Holmes ML, Corbin J, Vandenberg CJ, Alexander WS, Ng AP, Strasser A, Bouillet P, Sola-Visner M, Kile BT, Josefsson EC. BCL-2 is dispensable for thrombopoiesis and platelet survival. Cell Death Dis. 2015 Apr 16;6:e1721. doi: 10.1038/cddis.2015.97. PubMed PMID: 25880088; PubMed Central PMCID: PMC4650559.
- Lee BY, Han JA, Im JS, Morrone A, Johung K, Goodwin EC, Kleijer WJ, DiMaio D, Hwang ES. Senescence-associated beta-galactosidase is lysosomal beta-galactosidase. Aging Cell. 2006 Apr;5(2):187-95. PubMed PMID: 16626397.
- Rahikkala AT, Pereira S, Figueiredo PI, Passos M, Araújo A, Saraiva ML, Santos HA. Mesoporous silica nanoparticles for targeted and stimuli-responsive delivery of chemotherapeutics: a review. Adv Biosystems. 2018; 1800020. DOI: 10.1002/adbi.201800020
- Yanes RE, Tarn D, Hwang AA, Ferris DP, Sherman SP, Thomas CR, Lu J, Pyle AD, Zink JI, Tamanoi F. Involvement of lysosomal exocytosis in the excretion of mesoporous silica nanoparticles and enhancement of the drug delivery effect by exocytosis inhibition. Small. 2013 Mar 11;9(5):697-704. doi: 10.1002/smll.201201811. Epub 2012 Nov 14. PubMed PMID: 23152124; PubMed Central PMCID: PMC3767416.
- Ekkapongpisit M, Giovia A, Follo C, Caputo G, Isidoro C. Biocompatibility, endocytosis, and intracellular trafficking of mesoporous silica and polystyrene nanoparticles in ovarian cancer cells: effects of size and surface charge groups. Int J Nanomedicine. 2012;7:4147-58. doi: 10.2147/IJN.S33803. Epub 2012 Jul 31. PubMed PMID: 22904626; PubMed Central PMCID: PMC3418080.
- Mekaru H, Lu J, Tamanoi F. Development of mesoporous silica-based nanoparticles with controlled release capability for cancer therapy. Adv Drug Deliv Rev. 2015 Dec 1;95:40-9. doi: 10.1016/j.addr.2015.09.009. Epub 2015 Oct 3. Review. PubMed PMID: 26434537; PubMed Central PMCID: PMC4663124.
- Lozano-Torres B, Galiana I, Rovira M, Garrido E, Chaib S, Bernardos A, Muñoz-Espín D, Serrano M, Martínez-Máñez R, Sancenón F. An OFF-ON Two-Photon Fluorescent Probe for Tracking Cell Senescence in Vivo. J Am Chem Soc. 2017 Jul 5;139(26):8808-8811. doi: 10.1021/jacs.7b04985. Epub 2017 Jun 23. PubMed PMID: 28625064.
- Muñoz-Espín D, Rovira M, Galiana I, Giménez C, Lozano-Torres B, Paez-Ribes M, Llanos S, Chaib S, Muñoz-Martín M, Ucero AC, Garaulet G, Mulero F, Dann SG, VanArsdale T, Shields DJ, Bernardos A, Murguía JR, Martínez-Máñez R, Serrano M. A versatile drug delivery system targeting senescent cells. EMBO Mol Med. 2018 Sep;10(9). pii: e9355. doi: 10.15252/emmm.201809355. PubMed PMID: 30012580; PubMed Central PMCID: PMC6127887.