My name is Srinidhi Venkatesan Kalavai. I am a student at Boston University, majoring in Biomedical Engineering. As I grew up, a lot of my family were afflicted with age-related diseases such as Alzheimer’s Disease and cancer. Seeing the impact such diseases had not only on my grandparents but also on everyone in my family made me want to understand these diseases and contribute to curing them. The SRF Summer Scholars Program allows me to learn more about aging and the research being conducted to find ways to understand age-related diseases.
At Boston University, I work at Dr. Tsuneya Ikezu’s Laboratory for Molecular NeuroTherapeutics. The lab focuses its research on Alzheimer’s Disease (AD) pathology and its progression. As AD currently affects an estimated 5.4 million Americans, we want to understand the cause and progression of the disease. The aggregation of two proteins have been implicated in AD progression: tau and amyloid beta (Aß) (Winston et al. 2016). The accumulation of Aß is not well correlated to the clinical symptoms of AD, whereas research suggests that tau plays a critical role in the disease and that pathological tau species can be propagated by mouse exosomes in the mouse brain (Asai et al. 2015). Exosomes are cell-derived vesicles that participate in intracellular signaling and waste removal. A previous study from the lab showed that when mouse exosomes containing aggregated human tau were injected into mouse brains, exosomal tau was propagated into the hippocampus (Asai et al. 2015). The hippocampus is a part of the brain associated with long-term memory and formation of new memories. In AD, the hippocampus shrinks, resulting in memory loss in patients. We aim to demonstrate that human exosomes containing tau are propagated in a manner similar to mouse exosomes. By proving that tau can be propagated via human exosomes, we can elucidate a potential mechanism underlying AD progression. Additionally, by demonstrating that tau propagation is enhanced by the presence of Aß plaques, we can begin to understand the link between Aß and tau pathology in mouse models, as well as contribute to our knowledge of human pathology. Knowing the mechanism for AD pathology will provide pharmaceutical targets for combating Alzheimer’s Disease. I will be returning to this project in the fall.
Understanding TOR activation in Intestinal Stem Cell Response to Injury
Through the SRF Summer Scholars Program, I am working at The Buck Institute for Research on Aging at Dr. Henri Jasper’s lab. Under the direction of Dr. Samantha Haller, I will be studying the TOR pathway in intestinal stem cells of fruit flies to understand the effect of metabolism on stem cell function. The TOR pathway is involved in cell growth by regulating protein synthesis and metabolism, autophagy, transcription and ribosome biogenesis (Wullschleger et al., 2006).

Figure 1. Mammalian TOR pathway.
TOR consists of two complexes, TORC1 and TORC2. The overall effect of TORC1 activation is growth in cell size, while TORC2 controls the direction in which the cell grows. TOR activation is repressed by TSC1 and TSC2. TSC1 and TSC2 are regulated by insulin, stress and hypoxia factors, energy present in the cell and nutrients available to the cell. The Insulin pathway is well characterized but the other factors controlling TOR expression are unknown. Arrows represent activation, bars represent inhibition. (Figure taken from Wullschleger, Loewith and Hall, 2006)
The tissue homeostasis of the intestine is maintained by intestinal stem cells (ISCs), the only cells able to divide in the Drosophila midgut (Ohlstein & Spradling, 2007). Homeostasis refers to maintenance of tissue structure and function by balancing cell death, proliferation and differentiation. Upon their division, ISCs will produce two daughter cells. One of them will remain a stem cell (ISC) and the other will start to differentiate to form an enteroblast (EB). In ISCs, TOR is inhibited by high levels of TSC2, an upstream inhibitor of TOR. This repression of TOR is necessary for maintenance of ISC “stemness” (Kapuria, 2012). In EBs, TSC2 expression is suppressed, allowing an activation of TOR signaling. Depending on the levels of TOR activity, an EB will then give rise either to enterocytes (ECs) or to enteroendocrine (EE) cells. High levels of TOR in EBs result in differentiation into ECs, while relatively lower TOR levels results in EE formation (Kapuria, 2012). ECs form the epithelium of the intestine and are involved in absorption of nutrients, while EEs are intestinal endocrine cells and produce hormones.

Figure 2. The Drosophila gut and the different cell types present.
The crop is a storage organ and is used to store food that is ingested by the fly. The crop leads into the proventriculous(PV) and then the midgut. The drosophila midgut consists of the anterior midgut(AM), middle midgut(MM) and posterior midgut(PM). The posterior midgut contains ISCs which can divide to form EBs which further differentiate into ECs and EEs. The midgut leads into the hindgut(HG). Malphigian tubules(MT) (they perform the function of the kidneys in flies) are attached at the connection between the hindgut and the midgut. (Figure taken from Ayyaz & Jasper, 2013)

Figure 3. The intestinal stem cell proliferation and differentiation mechanism.
High TSC2 levels repress TOR in ISCs, maintaining the cell as a stem cell. The cell divides to form EBs. High levels of Delta/Notch(DI/N) signaling corresponds to high TOR levels in EBs which leads to EC formation, while low DI/N signaling corresponds to low TOR levels resulting in EE formation. (Figure taken from Kapuria et al. 2012)
Additionally, it has been shown in the muscle stem cells of the mouse that, in response to injury, quiescent stem cells (G0) (stem cells that are currently inactive and out of the cell cycle but have the capability to reenter the cell cycle when stimulated) can enter an alert state (Galert), which allows them to enter the cell cycle and repair the injury quickly. TOR activation seems to be essential for stem cells to enter this Galert phase, proliferate and allow a quick regeneration of the tissue. Similar results have been observed in the ISCs of flies (Rodgers et al., 2014).
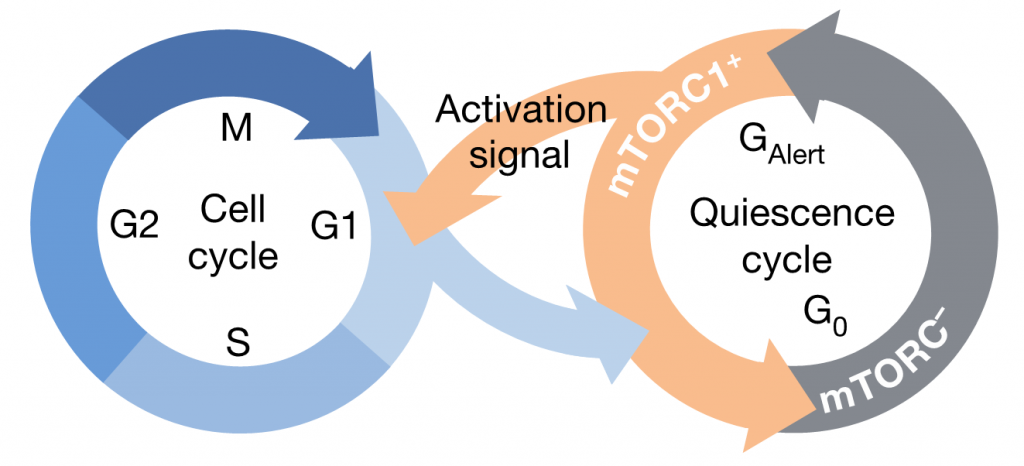
Figure 4. Model of the cell cycle in muscle stem cells of mice.
Therefore, the TOR pathway seems to be critical for both the proliferation and differentiation of stem cells. The TOR pathway is regulated by many different mechanisms such as insulin, glucose and ATP. It has been shown that nutrients can regulate TOR, but the exact molecular mechanism involved in regulating TOR is unknown (Guertin and Sabatini, 2007). Thus, the goal of this project is to better understand the molecular mechanisms that are responsible for TOR activation in ISC response to injury.
Future Plans:
Next semester, I will be returning to Dr. Tsuneya Ikezu’s laboratory to complete my project as well as my senior design project. Once I graduate, I plan on pursuing a PhD and further study aging and age-related diseases.
References:
Asai, Ikezu, Tsunoda, Medalla, Luebke, Haydar, Wolozin, Butovsky, Kugler and Ikezu. “Depletion of Microglia and Inhibition of Exosome Synthesis Halt Tau Propagation.” Nature neuroscience 18.11 (2015): 1584–1593. doi:10.1038/nn.4132
Ayyaz and Jasper. “Intestinal inflammation and stem cell homeostasis in aging Drosophila melanogaster.” Frontiers in Cellular and Infection Microbiology, 3, 98. (2013): Web. http://doi.org/10.3389/fcimb.2013.00098
Guertin, and Sabatini. “Defining the Role of mTOR in Cancer.” Cancer Cell 12.1 (2007): 9-22. doi 10.1016/j.ccr.2007.05.008.
Kapuria, Karpac, Biteau, Hwangbo, and Jasper “Notch-Mediated Suppression of TSC2 Expression Regulates Cell Differentiation in the Drosophila Intestinal Stem Cell Lineage.” PLoS Genetics 8(11) (2012): Web. doi:10.1371/journal.pgen.1003045
Ohlstein, and Spradling. “Multipotent Drosophila Intestinal Stem Cells Specify Daughter Cell Fates by Differential Notch Signaling.” Science 315.5814 (2007): 988-992. DOI: 10.1126/science.1136606
Rodgers, King, Brett, Cromie, Charville, Maguire, Brunson, Mastey, Liu, Tsai, Goodell, and Rando. “mTORC1 controls the adaptive transition of quiescent stem cells from G0 to GAlert” Nature 510 (2014): 393-396. doi:10.1038/nature13255
Winston, Goetzl, Akers, Carter, Rockenstein, Galasko, Masliah, and Rissman. “Prediction of Conversion from Mild Cognitive Impairment to Dementia with Neuronally Derived Blood Exosome Protein Profile.” Alzheimer’s & Dementia : Diagnosis, Assessment & Disease Monitoring 3 (2016): 63–72. https://doi.org/10.1016/j.dadm.2016.04.001
Wullschleger, Loewith, and Hall. “TOR Signaling in Growth and Metabolism.” Cell (2006): 471-484. doi 10.1016/j.cell.2006.01.016